Tech notes
Video-Rate Microwave Imaging for Concealed-Threat Detection
A flexible, modular imaging system can rapidly detect threats concealed under clothes or hidden in bags.
Public spaces such as mass transit systems, stadiums, and large arenas face the potential for high-impact security incidents because of the heavy foot traffic in those places and the ease with which pedestrians could conceal threat items. Real-time imaging technology that detects threat items hidden under clothing or
in bags can serve as a powerful tool in securing these vulnerable environments. Microwave imaging, unlike x-ray sensing, is well-suited to screening humans because it uses safe, non-ionizing radiation (similar to that of cellular phones or wireless routers). Furthermore, it offers far superior material penetration than does either terahertz or infrared sensing.
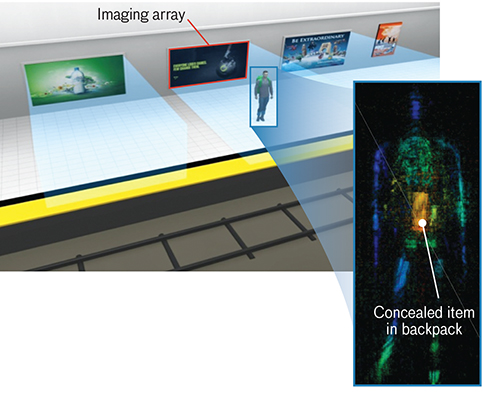
Microwave imaging systems have been successfully used in safeguarding air terminals. However, those systems require a screened subject to stop and pose for several seconds. In high foot-traffic environments, this disruption is unacceptable, highlighting a need for a more high-throughput solution.
MIT Lincoln Laboratory has developed a microwave imaging system that can operate at video rate (~10 Hz) to handle a constant stream of subjects. This system employs multistatic array sampling for the hardware-efficient realization of its required electrically large aperture, a fast imaging technique for video-rate image formation, and a modular design for flexible implementation. A proof-of-concept prototype has been constructed, and the design has been validated experimentally.
Operational Principles
The Laboratory's prototype system is an imaging radar aperture that can be placed on a wall or within a corridor to form microwave images of a subject as he or she passes by. Because microwave radiation penetrates clothing and bags but is mostly reflected by skin, concealed items are revealed in the images. It is envisioned that material-classification algorithms (currently under development at Lincoln Laboratory) will enable the creation of images that preserve privacy. These algorithms may be used, for example, to provide an operator with an image that only shows parts not identified as "skin."
The system is based on an antenna array that measures 0.5–1.5 m per side. The array's elements are coupled to a large switch matrix that is in turn coupled to
a radar transceiver and processor. The system interrogates a scene by switching to one transmit antenna and emitting
a low power (2–3 orders of magnitude weaker than a cellular phone transmission) microwave signal. It then captures signals reflected from the scene with one or more receive antennas. For faster acquisition, the system can probe the scene with multiple transmit antennas operating simultaneously on orthogonal waveforms (e.g.,
at different frequencies). The preceding process is repeated for many different transmit and receive antenna combinations at multiple frequencies to acquire all data needed to form an image of the scene. The acquisition takes just 10s of milliseconds to capture a subject in moderate motion. The full set of radar echoes is formatted and processed via synthetic aperture radar (SAR) reconstruction. Image formation can be aided by employing a range-finding camera to restrict the imaging area, thus reducing the processing load.
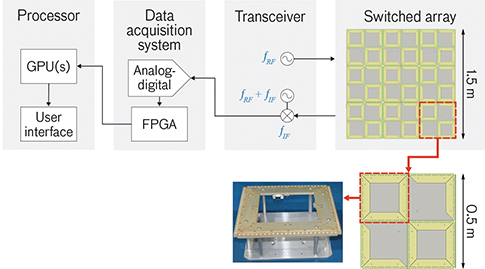
Key Design Aspects
Multistatic Array Sampling
One major challenge presented by the system is the need for a cost-effective electrically large array. For adequate illumination of a human subject, a relatively large (0.5–1.5 m) aperture is necessary. Using conventional SAR sampling techniques
(e.g., monostatic sampling, which requires colocated transmit and receive antenna elements) would demand arrays with extremely high numbers of antennas. For example, a monostatic aperture operating at 30 GHz would require about 40,000 antenna elements per 1 m2, making the system prohibitively costly. The prototype system employs multistatic sampling, for which transmit and receive antennas are not colocated and are not separated by a uniform distance. This paradigm allows for SAR sampling of a scene with far fewer antenna elements: only 480 transmit antennas and 480 receive antennas per
1 m2 at 30 GHz.
Fast Imaging Technique
The targeted application demands image reconstruction at video rates to handle the high foot traffic and to properly screen moving subjects. Additionally, it demands the use of an electrically large imaging aperture and a similarly large imaging domain. The required image reconstruction can be computationally intensive, and video-rate implementation on economical processors is a challenge. The backprojection technique is often used in microwave imaging, but its computational loads are prohibitive for this application. Fast Fourier transform
(FFT) imaging provides tractable loads for monostatic sampled arrays, but it is not readily compatible with highly thinned multistatic arrays. The Laboratory's system employs a low-overhead data correction that causes the multistatic-sampled data to approximate monostatic-sampled data and that allows the data to then be processed with FFT imaging for fast (2–3 orders of magnitude faster than backprojection) reconstruction on cost-effective computing hardware.
Modularity
The system is designed to be modular and flexible. It is configured as a set of identical 0.5 m × 0.5 m blocks. This arrangement allows for apertures of varying sizes to accommodate different use cases and to simplify the manufacture of the system. Because no microwave signals are exchanged between blocks, multiple blocks can be seamlessly integrated into the system.
Experimental Results
A partial aperture version of the system (0.25 m × 0.25 m) that operates from 24–28 GHz was constructed and tested. To sample the scene as a larger version of the array would, the system was placed on a mechanical scanner and moved
to multiple points (e.g., 6 × 6 positions for a 1.5 m scan) at which it sampled the scene with all transmit–to-receive element sets. Using this setup, the system formed high-fidelity three-dimensional microwave images in which concealed items were clearly revealed. A processor comprising four commercial off-the-shelf (COTS) graphical processing units (GPUs) was used to demonstrate that images of
a human-sized domain created from a
1.5 m × 1.5 m scan can be constructed in less than 0.1 seconds. This result illustrates that video-rate image formation is feasible on affordable COTS computing hardware.
Current efforts focus on scaling the system to a full 1.5 m x 1.5 m aperture. Then, capabilities testing and use-case studies will be conducted to inform possible roles of concealed item imaging technology in challenging security environments.
Posted November 2016
top of page