Shadowy Work
An array of telescopes could figure out what geosynch satellites are doing by studying their silhouettes.
Jane Luu and Leaf Jiang are chasing shadows. Specifically, the two members of Lincoln Laboratory’s Active Optical Systems group want to capture the shadows of geosynchronous satellites and examine them to see what they can learn about the satellites’ functions.
The U.S. Air Force tracks these satellites, which orbit at an altitude of 36,000 kilometers, but their telescopes see, essentially, blobs. It’s possible to tell where the satellites are but not what they’re doing. “Even the biggest telescope on Earth can only get a resolution of three meters, which is very poor by defense standards,” says Luu. If the satellite being observed is 10 m long, 3 m resolution will produce an image based on only three points.
Radar does a good job of imaging satellites that have discernible motion. But because geosynchronous satellites don’t move with respect to the Earth’s surface, and the newer ones don’t spin, there is no movement to produce the Doppler signals that radar needs to make out a shape.
Luu, a former astronomer, and Jiang, an electrical engineer, think the solution is to capture the shadows that satellites cast on the ground as stars pass behind them. Using a line of 150 separate 30 cm telescopes, they can create the equivalent of a single telescope with a 45 m aperture. The resulting half-meter resolution produces images sharp enough to get a clear silhouette of a satellite that will show its shape, where it’s pointing, and whether it’s carrying a telescope or other device—the sort of information that will give clues to the satellite’s function.
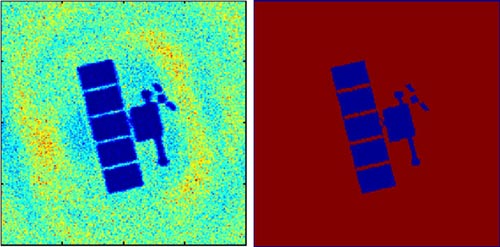 At left, a simulation made by 150 telescopes, each with a 30 cm aperture, in a 45-meter-long array, portrays shadow imaging of two geosynch satellites as produced by a star of magnitude 7.5. Compare the simulated image to the actual shapes of the satellites at right.
As a star passes behind a satellite, the light is diffracted around the edges, casting an array of ripples as the light waves interfere constructively and destructively. To reconstruct the image, the researchers measure the variations in intensity of the ripples, then run the data through an inverse Fourier transform, a standard processing algorithm. That’s the easy part. The hard part is catching the shadow in the first place.
Depending on the size of the satellite, a star passes behind it in a few milliseconds. To capture such a fleeting signal, the team uses avalanche photodiodes, which can detect single photons. The observations are designed to be made in blue wavelengths; this spectral selectivity screens out reflected sunlight from the satellites, which tends to comprise mostly yellow and red.
Luu and Jiang need to know with a fair degree of precision when a star will pass behind a satellite. Because a 10 m object in orbit casts a shadow that is only 15 m long, there’s not much margin for error. The researchers can use Air Force data to calculate the orbital position of a satellite fairly closely, but can wait months for a satellite and a star to line up. To solve this problem, they put the telescopes on a track, so that they can move them back and forth and line them up where they need to be. Luu and Jiang estimate that a 10-km-long track should serve most purposes, leading to one occultation per satellite per night.
“If you could build a 45 meter telescope on the ground, the image quality would be just as good as with our method—but no such telescope exists,” says Luu. Nor is it likely to. Such a telescope would be vastly more expensive than an array of 30 cm telescopes, which cost about $6000 apiece. And it would have to overcome the distortions caused by the weight of that large a mirror.
Scaling up the system should be a simple matter, Jiang says. “Just buy more telescopes and add them on.” He thinks it should be possible to get resolutions in the tens of centimeters by adding more telescopes, although that would also mean dealing with minor sources of distortion, such as chromatic dispersion, in which the atmosphere at higher latitudes spreads out different wavelengths of light.
While their aim is to track satellites for national defense purposes, the same set-up could be used to look for small objects, such as asteroids, within the solar system, as well as Earth-scale planets in other solar systems, where so far only Jupiter-sized bodies have been found. “Anything that goes in front of a bright source, you can find,” Luu says.
The Lincoln Laboratory team performed simulations showing that the idea should work, and is now aiming to demonstrate experimentally, possibly as early as September, using two telescopes. One or two telescopes will not produce an ideal image, but Luu and Jiang hope it will be enough to encourage a sponsor to endorse a full-scale project. Part of their work involves writing software to determine the satellite’s position and to automate the alignment of the telescopes. Once they get sponsorship, deploying an actual system would take about one year, according to Luu. “The concept is simple,” she says. “The actual implementation is hard.” “Although still easier than building a 45-meter telescope,” Jiang puts in. |