News
MIT Lincoln Laboratory wins four 2011 R&D 100 Awards
Awards recognize innovations in imaging technology, radar sensors,
computing, and biology
Four technologies developed at MIT Lincoln Laboratory were named 2011 recipients of R&D 100 Awards. Given annually by R&D Magazine, an international journal for research scientists and engineers, these awards recognize the 100 most technologically significant innovations introduced during the previous year. Recipients of R&D 100 Awards are chosen from hundreds of nominations by an independent panel of evaluators and the editors of R&D Magazine. Winners represent a broad range of technologies developed by industrial enterprises, government laboratories, and university research facilities.
The Laboratory's 2011 award winners are
- Airborne Ladar Imaging Research Testbed — an airborne laser radar that rapidly collects high-resolution three-dimensional imagery of wide-area terrains
- Multifunction Phased Array Radar Panel — panels of phased arrays exploit dual polarization and digital beamforming to provide efficient radar detection and tracking of aircraft and weather targets
- Parallel Vector Tile Optimizing Library — a real-time signal processing library that enables cross-platform portability of programs without sacrificing high performance
- Pathogen Analyzer for Threatening Environmental Releases Bioaerosol Identification System — a highly sensitive sensor that uses genetically modified white blood cells to rapidly detect and identify pathogens and toxins
Wide-area three-dimensional imaging
The Airborne Ladar Imaging Research Testbed (ALIRT) is an airborne laser radar (ladar) imaging system that provides both high-resolution, three-dimensional (3D) views of terrain from altitudes up to 9000 meters, as well as decimeter (10 cm) accuracy from altitudes of 3 km. ALIRT's high data-collection rates (7 to 12 times larger than any other available ladar system) and high operational altitudes (4 times that of other systems) enable unparalleled functionality. The system can obtain data over a wide range of terrain, including mountains and deep valleys, and from distances that permit increased operational security.
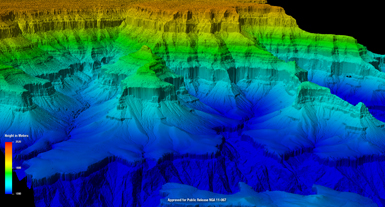
ALIRT produces 3D images of wide areas quickly —scanning 800 to 2200 km2 per hour—and has numerous applications for military and civilian users. ALIRT's ability to collect 3D information from such great altitudes, coupled with its unsurpassed data-collection rate, allows the system to obtain large amounts of data in a single pass. Maps generated by ALIRT reveal details of both natural and man-made structures.
"The ALIRT team was most fortunate in being given the opportunity of exploiting two earlier Lincoln Laboratory R&D-100-awarded technologies—the Geiger-mode APD [avalanche photodiode] detector arrays and solid-state microchip lasers—to develop this high-performance airborne lidar system to aid current military efforts," says program manager Robert Knowlton.
ALIRT data can be used to obtain human-scale imagery from standoff distances, distinguish nearby surfaces (e.g., to assess road and bridge trafficability or helicopter landing zones), detect objects obscured by foliage or camouflage, evaluate geometrical change, collect country-sized reference maps, and build detailed elevation models on an unparalleled scale. In January 2010, ALIRT provided data for maps used to evaluate conditions in post-earthquake Haiti, and during deployment to Afghanistan since October 2010, it has collected imagery data on more than 150 flights.
The ALIRT system was developed by a team led by Knowlton and comprising members from the ISR and Tactical Systems, Engineering, and Advanced Technology Divisions: Joseph Adams, Ross Anderson, Leonas Bernotas, Kenneth Burkett, Brandon Call, Robert Carlson, David Ceddia, Vincent Cerrati, Paul Consalvo, Jack Duston, Robert Freehart, Dale Fried, Robert Hatch, Richard Heinrichs, Alex McIntosh, Joseph McLaughlin, Christopher McNeil, James Mooney, Michael O'Brien, Frank Perry, Brian Player, Ryan Poplin, Christopher Reichert, John Shelton, Tina Shih, Luke Skelly, Prem Ramaswami, Daniel Stremeckus, Paul Tirone, Alexandru Vasile, Berton Willard, Alexander Wilson, and John Zayhowski. In addition, the team was supported by pilots Robert Keller and Bryan Williams of Sunshine Aero Industries.
Next-generation solution for providing weather and aircraft surveillance
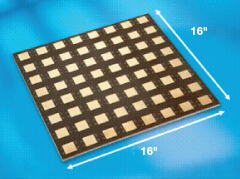
The Multifunction Phased Array Radar (MPAR) concept was developed as a replacement for earlier-era radar networks currently supplying air traffic and weather surveillance. An MPAR system has no moving parts and electronically shapes and steers its radar beam. This variation from current civilian radar systems, which use rotating antennas, provides a beam agility that enables increased resolution and faster full-volume scan rates, as well as the ability for one radar unit to perform multiple weather and atmospheric surveillance tasks.
"The next generation of FAA [Federal Aviation Administration] radars will likely use active phased arrays to provide improved air safety and weather forecasting. The MPAR panel combines DoD [Department of Defense] multifunction radar concepts with commercial wireless manufacturing techniques to meet performance and cost goals," says Lincoln Laboratory program manager Jeffrey Herd.
The MPAR's active electronically scanning phased-array antenna panel is the fundamental building block of an MPAR system. The phased-array panels function coherently to radiate and receive pulses of radar energy that are used to detect, locate, and track both aircraft and weather targets. MPAR is able to accomplish both types of surveillance modes by utilizing multiple simultaneous beam clusters that provide the flexibility to optimally manage the time and energy resources of the multimission system. One advantage of such flexibility is a reduction in the total number of ground-based radar sensors needed for weather and primary aircraft surveillance.
Under sponsorship of the FAA, the MPAR panel was jointly developed by Lincoln Laboratory and M/A-COM Technology Solutions. The developers from the Laboratory are members of the ISR and Tactical Systems and the Homeland Protection and Air Traffic Control Divisions, led by program manager Jeffrey Herd: Glenn Brigham, John Cho, David Conway, Sean Duffy, Sean Tobin, and Mark Weber. Team members from M/A-COM are Douglas Carlson, Christopher Weigand, and Daniel Curcio.
Software portability for real-time parallel processing
The Parallel Vector Tile Optimizing Library (PVTOL) is a portable C++ software library designed for developing embedded signal processing applications that increasingly require the use of programmable parallel processors for their computationally intensive tasks. PVTOL addresses a major technical hurdle for software developers: how to write programs at high level while still achieving high performance and preserving the portability of the code across parallel computing hardware platforms.
"PVTOL simultaneously provides performance, portability, and programmer productivity benefits for real-time parallel signal processing software development," says Edward Rutledge, one of the library's developers.
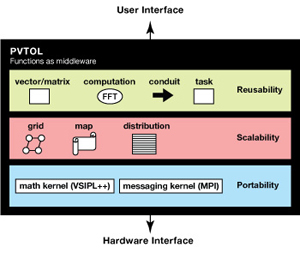
PVTOL enables signal processing algorithm code to be written at a high level by using task and data objects that insulate the application from details of how those objects are mapped to the parallel architecture or how they synchronize and communicate. PVTOL programs are scaled easily by changing the maps of the distributed objects. Mapping is specified separately from the algorithm code, so that code need not be changed to adapt to the number of processing elements being used. Because the PVTOL application programming interface is constant across platforms, developers do not have to change the application code when they port the application to a new hardware platform.
PVTOL was developed by a team from the Laboratory's Embedded and High Performance Computing Group: James Daly (lead developer), Nadya Bliss, Robert Bond, Karen Eng, Ryan Haney, Hahn Kim, Jeremy Kepner, Scott Lacroix, Sanjeev Mohindra, Edward Rutledge, and Glenn Schrader.
Rapid identification of pathogens
The Pathogen Analyzer for Threatening Environmental Releases (PANTHER) Bioaerosol Identification System collects and identifies pathogens in the air in less than two minutes by using a cell-based technology known as Cellular Analysis and Notification of Antigen Risks and Yields (CANARY). CANARY utilizes B cells genetically engineered to produce light within seconds of binding to specific pathogens of interest, including the bacteria that cause anthrax and plague. PANTHER analyzes an air sample for up to 48 different bioagents by spinning small drops of CANARY cells stored inside a disk onto aerosol particles collected directly from the air into special structures at the edge of the disk. After the cells mix with the particles, the resulting light from each drop is measured to determine which pathogens are present. All of this is accomplished automatically within two minutes in a 1 cubic foot, 37 pound box that is as easy to load and operate as a DVD player.
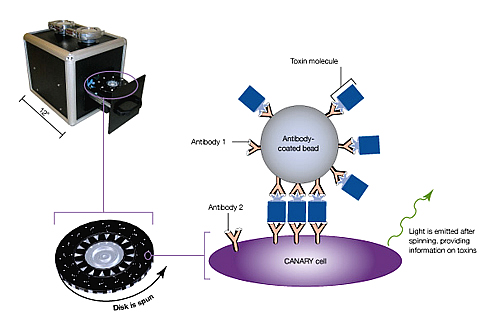
"The unique combination of speed and sensitivity enabled by PANTHER sensors offers the ability to identify many pathogens in time to protect individuals in an infected area rather than just wait to treat them after they are sick," says James Harper, one of the lead developers of the system.
PANTHER and the underlying CANARY technology were developed by a team from the Advanced Technology, Homeland Protection and Air Traffic Control, and Engineering Divisions. Harper, Joseph Lacirignola, and Richard Mathews led the development of the portable PANTHER prototype, and Shaun Berry, Catherine Cabrera, John D'Angelo, Mark Hollis, Frances Nargi, John O'Fallon, Lalitha Parameswaran, Martha Petrovick, Todd Rider, Eric Schwoebel, Timothy Stephens, and Trina Vian made significant contributions to the underlying CANARY technology and sensor hardware that made PANTHER possible.
Past awards
In 2010, Lincoln Laboratory received R&D 100 Awards for the digital-pixel focal plane array, Geiger-mode avalanche photodiode detector focal plane array, miniaturized RF four-channel receiver, runway status light system, and superconducting nanowire single-photon detector. The Laboratory received an R&D 100 Award in 1998 jointly with Cyra Technologies and the Los Alamos National Laboratory for the Cyrax Portable, 3D Laser-Mapping and Imaging System. A 1995 award was presented to the Laboratory for the GPS-squitter technology, a technique that uses GPS position data for automatic dependent surveillance of aircraft.
Posted August 2011
top of page